PROVIDENCE, R.I. — Few things are more frustrating for remote workers than the moment a wireless signal suddenly drops from their laptops. In an instant, their video call freezes, emails won’t send, and they’re left frustrated and disconnected. We’ve all been there, and it’s a problem that’s only going to get worse as more and more devices compete for limited bandwidth.
But what if there was a way to boost wireless capacity and speed, even in the most congested environments? Enter the world of terahertz (THz) wireless communication.
Terahertz waves, which sit between microwaves and infrared light on the electromagnetic spectrum, offer a tantalizing solution to our wireless woes. With frequencies ranging from 100 GHz to 10 THz, they have the potential to carry vast amounts of data – think high-definition video, virtual reality, and instant downloads – at speeds far exceeding our current networks.
However, harnessing the power of THz waves comes with its own set of challenges. One major hurdle is signal blockage. Because THz waves are so short (on the order of millimeters), they’re easily disrupted by obstacles like walls, furniture, and even people. This is a big problem for indoor networks, where users and objects are constantly moving around.
Now, a team of researchers from several U.S. universities may have found a way to bend THz beams around obstacles, opening up new possibilities for robust, high-speed wireless networks. According to study author Daniel M. Mittleman of Brown University, it could clear the biggest hurdle in the jump to 6G networks.
In a recent study published in the journal Communications Engineering, the researchers demonstrate a novel approach to engineering THz wavefronts that can follow curved trajectories. This allows the waves to essentially curve around obstacles, maintaining a strong link between the transmitter and receiver.
The key to their approach lies in the unique properties of the electromagnetic near field. In wireless communication, we typically think in terms of far-field links, where the transmitter and receiver are separated by many wavelengths. At these distances, the waves behave like rays traveling in straight lines.
But in the near field – the region close to the transmitter, within about one wavelength – the story is different. Here, the wavefronts are curved, and their behavior is governed by complex diffraction patterns rather than simple ray optics.
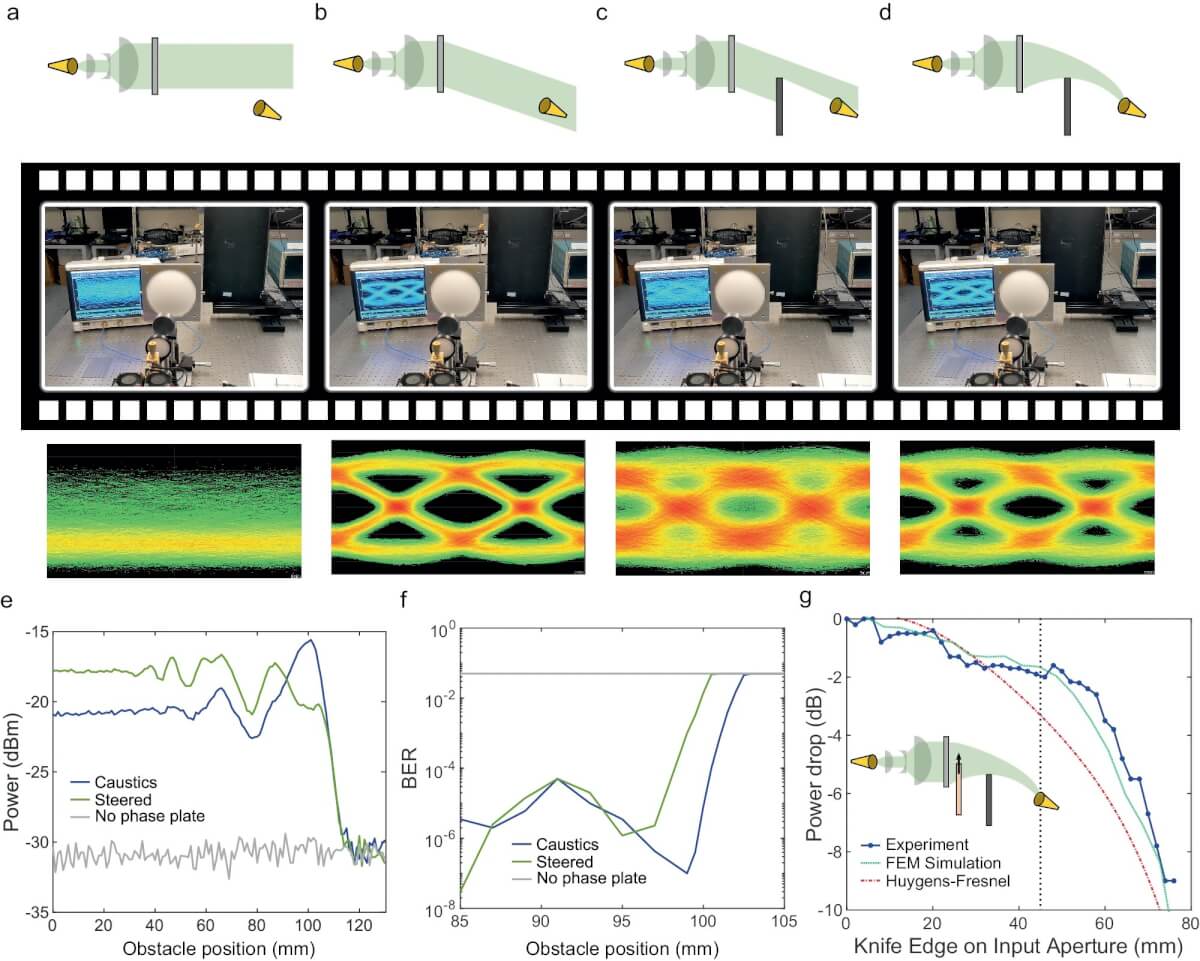
The researchers realized that, at THz frequencies, many realistic indoor scenarios would involve near-field links, with users located just meters from the transmitter. By carefully shaping the wavefront emitted by the transmitter, they found they could create “self-accelerating beams” that follow curved paths as they propagate through the near field.
To understand how this works, imagine a line of people passing a bucket of water down the line. Each person represents a point on the wavefront, and the bucket is the signal. Now, instead of passing the bucket straight down the line, each person hands it off at a slight angle. The result is a curved path that can wrap around obstacles.
The researchers demonstrated this principle using specially designed “phase plates” and “metasurfaces” – essentially, flat surfaces etched with intricate patterns that can manipulate the phase of the waves as they pass through. By encoding the right pattern, they were able to generate THz beams that followed parabolic trajectories, neatly avoiding a metal obstacle placed in their path.
Of course, there’s more to a wireless link than just steering the beam. The researchers also had to ensure that enough signal power reached the receiver to maintain a high data rate. They developed a new model for calculating link budgets in the near field, showing that self-accelerating beams can outperform traditional Gaussian beams at short ranges.
They also investigated the bandwidth limitations imposed by the curved beam paths. Because different frequencies travel at slightly different speeds, the beam can experience a “rainbow effect,” with different colors (frequencies) arriving at different times and places. The tighter the beam curvature, the more severe this effect. However, the researchers showed that even with this limitation, near-field links can support bandwidths of tens to hundreds of gigahertz – more than enough for ultra-high-speed data.
To put their concept to the test, the team set up a communication link at 200 GHz and used it to transmit data at speeds up to 1 gigabit per second. When a metal plate was moved into the beam path, simulating a sudden obstruction, the link maintained a low error rate by switching to a curved beam that wrapped around the obstacle.
While there’s still much work to be done to translate these results into practical wireless networks, the study offers a compelling glimpse into the future of THz communications. By leveraging the unique properties of near-field wavefronts, we may one day enjoy seamless, lightning-fast connectivity, even in the most challenging environments.
So the next time you’re struggling with a spotty wireless signal, take heart. The solution may be just around the bend – literally.
StudyFinds Editor-in-Chief Steve Fink contributed to this report.